Answer:
(a) By small angle approximation, we have;
F = -2×T×Δy/l
(b)

Step-by-step explanation:
(a) The diagram shows the mass, M, being restored by two equal tension, T acting on the elastic strings l, such the restoring force, F acts along the path of motion of the mass, with distance Δy
Therefore, the component of the tension T that form part of the restoring force is given as follows;
Let the angle between the line representing the extension of the elastic strings T and the initial position of the string = ∅
Then we have;
String force,
= T×sin∅ + T×cos∅ + T×sin∅ - T×cos∅ = 2×T×sin∅
Whereby the angle is small, we have;
sin∅ ≈ tan∅ = Δy/l
Which gives;
= 2×T×sin∅ = 2×T×Δy/l (for small angles)
Restoring force F =
= -2×T×Δy/l
F = -2×T×Δy/l
(b) Given that the the tensions do not change appreciably as the mass, M, oscillates from Δy we have;
By Hooke's law, F = -k×x
Whereby Δy corresponds to the maximum displacement of the mass, M from the rest position, which gives;
Which gives;
F = M×a = -k×Δy
a = -k×Δy/M
d²(Δy)/dt² = -k×Δy/M
When we put angular frequency as follows;
ω² = k/M
We get;
d²(Δy)/dt² = -ω²×Δy
Which gives;
Δy(t) = A×cos(ωt + Ф)
The angular frequency is thus, ω = √(k/M)
Period of oscillation = 2·π/ω = 2·π/√(k/M)
The frequency of oscillation, f = 1/T = √(k/M)/(2·π)
Where:
k = 2·T/l, we have;
f = √(k/M)/(2·π) = √(2·T/l)/m)/(2·π)
The frequency of oscillation is given as follows;
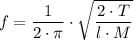